Quantum harmonic oscillator
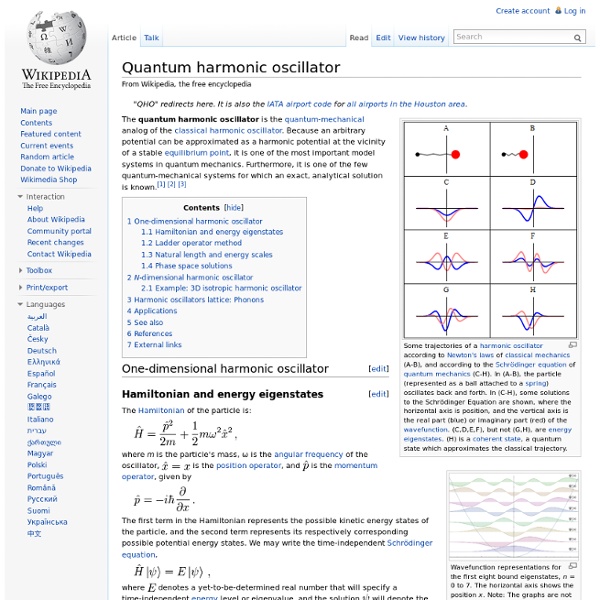
Some trajectories of a harmonic oscillator according to Newton's laws of classical mechanics (A-B), and according to the Schrödinger equation of quantum mechanics (C-H). In A-B, the particle (represented as a ball attached to a spring) oscillates back and forth. In C-H, some solutions to the Schrödinger Equation are shown, where the horizontal axis is position, and the vertical axis is the real part (blue) or imaginary part (red) of the wavefunction. The quantum harmonic oscillator is the quantum-mechanical analog of the classical harmonic oscillator. One-dimensional harmonic oscillator[edit] Hamiltonian and energy eigenstates[edit] Wavefunction representations for the first eight bound eigenstates, n = 0 to 7. Corresponding probability densities. where m is the particle's mass, ω is the angular frequency of the oscillator, is the position operator, and is the momentum operator, given by One may write the time-independent Schrödinger equation, The functions Hn are the Hermite polynomials, and
Mehler kernel
In mathematics and physics, the Mehler kernel is the fundamental solution, or non-relativistic propagator of the Hamiltonian for the quantum harmonic oscillator. Mehler (1866) gave an explicit formula for it, called Mehler's formula. The Mehler kernel provides the fundamental solution for the most general solution φ(x, y; t) to Specifically, Mehler's kernel is By a simple transformation, this is, apart from a multiplying factor, the bivariate Gaussian probability density given by When t=0 variables x and y coincide resulting in the formula The bivariate probability density can be written as an infinite series involving the one-dimensional probability densities and Hermite polynomials of x and y.
Mock modular form
In mathematics, a mock modular form is the holomorphic part of a harmonic weak Maass form, and a mock theta function is essentially a mock modular form of weight 1/2. The first examples of mock theta functions were described by Srinivasa Ramanujan in his last 1920 letter to G. H. History[edit] "Suppose there is a function in the Eulerian form and suppose that all or an infinity of points are exponential singularities, and also suppose that at these points the asymptotic form closes as neatly as in the cases of (A) and (B). Ramanujan's original definition of a mock theta function, from (Ramanujan 2000, Appendix II) Ramanujan's 12 January 1920 letter to Hardy, reprinted in (Ramanujan 2000, Appendix II), listed 17 examples of functions that he called mock theta functions, and his lost notebook (Ramanujan 1988) contained several more examples. Ramanujan associated an order to his mock theta functions, which was not clearly defined. Definition[edit] Fix a weight k, usually with 2k integral.
Harmonic series (mathematics)
In mathematics, the harmonic series is the divergent infinite series: The fact that the harmonic series diverges was first proven in the 14th century by Nicole Oresme,[1] but this achievement fell into obscurity. Proofs were given in the 17th century by Pietro Mengoli,[2] Johann Bernoulli,[3] and Jacob Bernoulli.[4] The harmonic series is counterintuitive to students first encountering it, because it is a divergent series though the limit of the nth term as n goes to infinity is zero. Because the series gets arbitrarily large as n becomes larger, eventually this ratio must exceed 1, which implies that the worm reaches the end of the rubber band. Another example is: given a collection of identical dominoes, it is clearly possible to stack them at the edge of a table so that they hang over the edge of the table without falling. There are several well-known proofs of the divergence of the harmonic series. for every positive integer k. The harmonic series diverges very slowly. where The series
Zonal spherical harmonics
In the mathematical study of rotational symmetry, the zonal spherical harmonics are special spherical harmonics that are invariant under the rotation through a particular fixed axis. The zonal spherical functions are a broad extension of the notion of zonal spherical harmonics to allow for a more general symmetry group. On the two-dimensional sphere, the unique zonal spherical harmonic of degree ℓ invariant under rotations fixing the north pole is represented in spherical coordinates by where Pℓ is a Legendre polynomial of degree ℓ. , where x is a point on the sphere representing the fixed axis, and y is the variable of the function. In n-dimensional Euclidean space, zonal spherical harmonics are defined as follows. to be the dual representation of the linear functional in the finite-dimensional Hilbert space Hℓ of spherical harmonics of degree ℓ. for all Y ∈ Hℓ. Relationship with harmonic potentials[edit] where is the surface area of the (n-1)-dimensional sphere. Properties[edit] If Y1,...
Related:
Related: